Research
Current projects:
Cellular midbrain mechanisms
Feedback circuits
Multimodal interactions
Development of neuronal networks
Future projects:
In-Vivo-Recordings
Optical imaging Experiments
Uncaging-Experiments
Cellular mechanisms
The basic units of information processing in the brain are neurons. Based on their dendritic organization, we attempt to gain a mechanistic understanding of single neuron input processing. We mostly use living slices of brain tissue (brain slices) and apply whole-cell patch recordings, circumscribed electrical stimulations with up to ten bipolar stimulus electrodes, and pharmacology to work out the cellular processing of defined input patterns. In combination with information on the afferent sources of a given cell, the transmitters and modulators involved, and the efferent targets we then model the cellular response; the predictions gathered with these models are then again validated in the slice preparation, until we have obtained a thorough understanding of a given cell’s physiology.
For most of our studies, we use the avian midbrain or, more specifically, the optic tectum as a model system. This structure offers a variety of advantages for the study of cellular mechanisms. First, afferents from the sensory pathways form topographical maps of the sensory environment, constructing a multimodal map of sensory space in the tectum Second, the visual afferents enter the optic tectum via the superficial layers before curving down to terminate in the upper 7 layers; this enables us to deliver patterned electrostimulation to the input layers, thus mimicking visual patterns on the retina. The anatomy of the optic tectum has been analysed thoroughly since the days of Ramòn y Cajal, and for all of the tectal layers there is detailed information available on the input structures, the neurotransmitters and modulators involved, the cell types found in the layer, the morphology of these neurons, and their efferent target structures throughout the brain. This wealth of information allows us to target specific neuronal types in a given layer repetitively and thus analyse their physiology to a high degree. In addition, the retino-tectal projection in birds has been a model system for the analysis of developmental processes such as map formation; thus, a detailed picture of the molecular cues defining tectal layers and positions is available and further aids in the targeting of specific neurons. Taken together, it is possible to construct a very detailed wirinig diagram of the optic tectum, which facilitates the analysis of a given cell type.
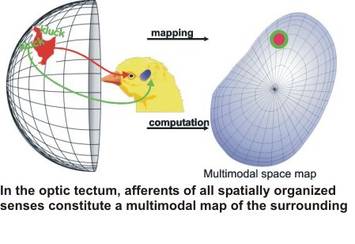
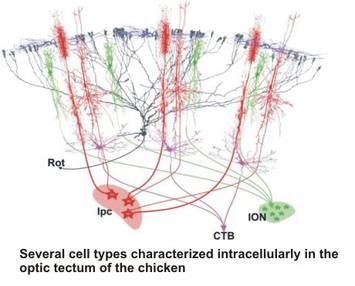
Feedback circuits
The analysis of specific cell types is only the starting point for our quest to understand small neuronal networks, their physiology and their successive integration into larger networks in the brain. As an example, we are currently studying the feedback circuit of the optic tectum with the nuclei of the isthmic area. The latter nuclei are well-defined, compact structures in the midbrain with an almost exclusive in- and output relation to the optic tectum. This system has a comparable layout in most vertebrates, and has been studied in detail in various avian species. The the anatomical layout in birds is intriguing and suggests a function as a winner-takes-all circuit, possibly subserving the role of disambiguating the tectal saliency map which is fundamental to bottom-up models of attention. Since parts of the tecto-isthmo-tectal networks remain intact in a slice preparation of the midbrain, we have the unique opportunity to study the physiology and the temporal dynamics of a feedback circuit in a slice preparation, with all the advantagees of the in-vitro approach (ease of whole-cell patch recording, targeting of neurons under visual control, selective electrostimulation and pharmacological and microsurgical manipulation options).
The development of cellular interactions in the nervous system rely heavily of molecular gradients and cell-cell interactions through a plethora of membrane-bound molecules. The molecular cues that define parts of the tecto-isthmo-tectal networks (e.g., R-Cadherin) have been analysed in combined tracing/immunocytochemical studies. This knowledge offers up the possibility of manipulating the expression of specific factors during development (e.g., through RNAi) that could result in an altered layout of the feedback network. This in turn would allow us to investigate our concepts of the role of a given nucleus in the complex feedback loop directly, either in the slice or even in the intact behaving animal.
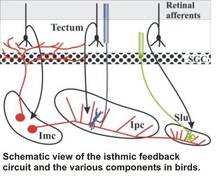
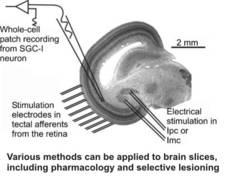
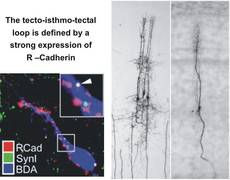
Multimodal interactions
The multimodal map of space in the optic tectum is assembled during development and is dominated by the visual system which acts as a master map for the other sensory afferents. Thus, manipulations in the visual map results in plastic events leading to the alignment of other (e.g., the auditory map) with the new visual topography. This process has been worked out in a series of experiments in barn owls by Eric Knudsen, and can probably also be extended to the other spatial sensory afferents such as somatonsensory, lateral line, or infrared vision. How the discrepancy in the sensory maps is read out and how this information leads to the plasticity in the afferent pathways, is still under debate. With intracellular methods, we have characterized the development of the auditory input to the optic tectum, and have identified a cell type in the tectum that might be the source of the error signal fed back into the auditory pathway. At the moment, we are investigating the multimodal midbrain network with a combination of intracellular labeling, patch physiology, tracing and immunocytochemistry, to get a deeper insight into the cellular mechanisms of multimodal alignment.
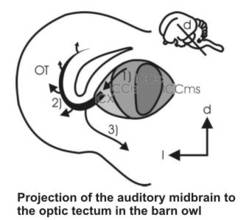
Development of neuronal networks
Neuronal networks have to be wired during embryonic development. This is especially pressing for precocial animals such as the chick, since the hatchling does not have a nesting phase where the fine-tuning of sensory input and cellular computation can occur. Thus, the determination of the neuronal networks largely has to be coded genetically and has to interact in a complex manner with molecular cues and electrical patterns of excitation prior to hatching. Over the years, we have analysed the dendritic development of several cell types in the optic tectum of chicken with and without embryonal manipulation (such as removal of the eye anlage early in development). To carry this analysis further, we have established a cell culture of chicken neurons from both the optic tectum and the auditory brainstem, and developed a method to sort neurons through magnet-based immunocoupling. We have studied the development of cellular characteristics under culture conditions, and delineated factors that control these steps. As a side aspect, we have collaborated with electrical engineers to eventually build a biohybrid system where we want to study the interplay of electrical stimulation and intracellular processes. This line of work also aims at reconstructing a given neuronal circuit in a biohybrid approach
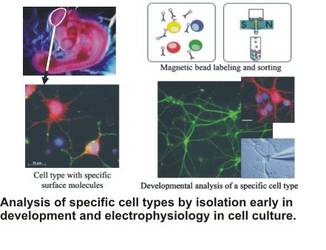
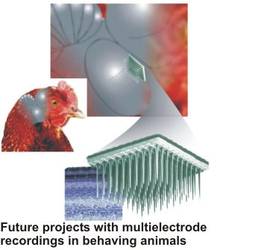
-Vivo-Recordings
After taking over the chair of zoology at the TUM, I will extend my research to work on intact animals. I want to pursue several projects in this direction: First, I want to record from the optic tectum of freely behaving animals with multielectrode arrays, to get definite data on the input streams from the retina that reach the optic tectum. These patterns of input will then be fed with stimulation electrode arrays into the tectal slice, to see whether the application of a “natural” input changes the behaviour of the neurons that we have analysed before in the “silent” slice preparation. Also, these recordings will allow us to put our hypotheses to a critical test, since from our slice studies of motion-detecting cells and feedback circuits we have clear predictions of the physiology in a given natural situation.
Optical imaging Experimente
The temporal dynamics of the feedback circuit with the isthmic nuclei can only be studied to a certain degree with single-electrode recordings in a slice preparation. We have thus started to study the temporal aspects with optical imaging methods in the slice, where we will use voltage-sensitive dyes to analyse the spread of excitation throughout the network and its components. These experiments will be carried out in a joint approach together with Prof. Schemann of the TU Munich (Chair of Human Biology).
Uncaging-Experiments
While patterned electrical stimulation in the midbrain slice can reveal the dendritic computation in some cell types, this technique is not adequate for neurons where the input structures are either obscure or not clustered enough to allow for a definite stimulation and to exclude the direct stimulation of the cell in question. We have thus started to establish an uncaging-setup, where the slice is incubated in transmitter which is caged with a molecular cage, that can be destroyed with a light impulse at a given position and a given time point. With this technique, we hope to map out the response of the neuron to input at different dendrites.